Factors Influencing the Solubility of Drugs
Upon completion of this exercise, you should be able to:
- Define solubility, miscibility, salting-in, salting-out, complex ion formation, dielectric constant, and pHp.
- Identify factors that affect drug solubility.
- Explain the effects of temperature, multiple solutes, pH, and solute/solvent polarities (dielectric constant) on drug solubility.
- Describe the fundamental relationship between product formulation, drug solubility, and drug absorption, bioavailability, and therapeutic efficacy.
- Describe two (2) approaches to optimizing drug solubility.
- Determine the pHp of a weak acid or base for a given concentration.
- Given necessary experimental data, calculate the dielectric constant needed to solubilize a compound.
One of the primary physicochemical considerations in preparing pharmaceutical solutions is the solubility of the drug in a suitable solvent. Solubility may be defined as the maximum concentration of a substance that may be completely dissolved in a given solvent at a given temperature and pressure. When both solute and solvent are liquids, the term miscibility rather than solubility may be used to describe the affinity between the liquids.
The solubility of a substance may be described in a variety of ways. The USP/NF generally expresses the solubility in terms of the volume of solvent required to dissolve 1 gram of the drug at a specified temperature (e.g., 1 g ASA in 300 ml H2O, 5 ml ethanol at 25°C). Other references may use more subjective terms to describe solubility, such as those given in the following table from Remington’s.
Descriptive terms | Parts of solvent needed for 1 part solute |
---|---|
Very soluble | < 1 |
Freely soluble | 1-10 |
Soluble | 10-30 |
Sparingly soluble | 30-100 |
Slightly soluble | 100-1000 |
Very slightly soluble | 1000-10,000 |
Practically insoluble or insoluble | > 10,000 |
Liquids which form a homogeneous system when mixed in any proportion are said to be miscible (e.g., water and ethanol). Those in which only certain volume ratios produce homogeneous mixtures are said to be miscible in certain proportions (e.g., water and chloroform). Immiscible liquids will not produce a homogeneous solution in any proportions (e.g., water and olive oil).
The aqueous solubility of all drugs is of interest to us, since it is only in the form of an aqueous solution that a drug can be absorbed into the general circulation to exert a therapeutic effect.
The solubility of any substance is a function of temperature. Most substances are endothermic, absorbing heat in the process of dissolution. For these substances, an increase in temperature results in an increase in solubility. A few substances, such as calcium hydroxide and sodium carbenicillin, are exothermic and give off heat in the process of dissolution. The solubility of such substances would decrease with an increase in temperature. The application of this aspect of solubility is of limited use to us, since pharmaceutical solutions must be administered at or near room or body temperature. It is more a factor to be considered for product storage than for formulation.
The aqueous solubility of nonelectrolytes is nearly always affected in some way by the addition of an electrolyte. Salting-out is the precipitation of organic solutes from aqueous solution by the addition of an electrolyte or salt. This is attributed to competition between solute molecules for the solvent and is dependent upon the size and valence of the ion. Salting-in is the increase in solubility of an organic solute upon addition of an electrolyte. The mechanism of this phenomenon is poorly understood and it is rarely encountered. An example is with the group of proteins called globulins which are more soluble in dilute salt solutions than in water. Complex ion formation occurs when an insoluble solute reacts with a soluble substance to form a soluble complex. An example is the addition of the soluble potassium iodide (KI) to the insoluble iodine molecule (I2) to form a soluble triiodide complex (KI3).
According to the Henderson-Hasselbach equation, the relationship between pH, pKa, and relative concentrations of an acid and its salt is as follows:
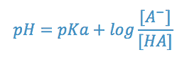
where [A–] is the molar concentration of the salt (dissociated species) and [HA] is the concentration of the undissociated acid. When the concentrations of salt and acid are equal, the pH of the system equals the pKa of the acid. As the pH decreases, the concentration of the molecular acid increases and that of the salt decreases. This has some interesting implications regarding the aqueous solubility of the acid, since the undissociated form is much less soluble than its salt. Of further interest, therapeutically, is the fact that it is the undissociated acid (HA) that more readily penetrates biological tissues to exert a therapeutic effect. Thus, in formulating the product, some balance must be struck between the more soluble salt form and the biologically active acid and factors other than pKa and pH must be considered (e.g. safety and comfort).
Changes in solubility brought about by alterations of solvent pH can be predicted by the pHp equation. The pHp is the pH below which an acid or above which a base will begin to precipitate.
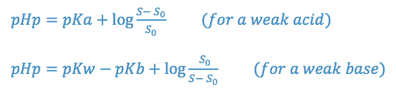
where,

e.g., Calculate the pHp of a 1% sodium phenobarbital solution.
From Merck Index:
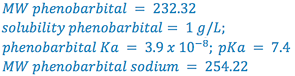



(i.e., 1% phenobarbital will precipitate at or below a pH of 8.3)
Solute and Solvent Structure/Polarity
Solute molecules are held together by certain intermolecular forces (dipole-dipole, induced dipole-induced dipole, ion-ion, etc.), as are molecules of solvent. In order for dissolution to occur, these cohesive forces of like molecules must be broken and adhesive forces between solute and solvent must be formed.
The solubility of a drug in a given solvent is largely a function of the polarity of the solvent. Solvents may be considered polar, semi-polar or non-polar. Polar solvents will dissolve ionic and other polar solutes (i.e. those with an asymmetric charge distribution [like dissolves like]), whereas, non-polar solvents will dissolve non-polar molecules. Semi-polar solvents (eg. alcohols and ketones) may induce a certain degree of polarity in non-polar molecules and may thus act to improve the miscibility of polar and non-polar liquids. The relationship between polarity and solubility may be used in practice to alter the solubility of a drug in a pharmaceutical solution.
One approach is to alter the polarity of the solute by shifting it between its molecular (undissociated) and ionic (dissociated) states. A shift toward the ionic form improves solubility of the solute in water and other polar solvents. A shift toward the molecular species improves solute solubility in non-polar solvents. Such shifts may be produced by altering the pH of the solution (or using the salt form of the compound).
Another approach is to mix solvents of different polarities to form a solvent system of optimum polarity to dissolve the solute. Such solvents must, obviously, be miscible. This method is referred to as solvent blending or cosolvency and uses the dielectric constant as a guide to developing the cosolvent system. Since many solvents may be toxic when ingested, most solvent blends are limited to mixtures containing water, ethanol, glycerin, propylene glycol, polyethylene glycol 400 or sorbitol solution. The list is somewhat expanded for solutions for external application.
The dielectric constant (δ) of a compound is an index of its polarity. A series of solvents of increasing polarity will show a similar increase in dielectric constant.
Compound | Dielectric constant, δ, @ 20°C |
---|---|
N-methylformamide | 190 |
Water | 80 |
Sorbitol Solution USP (70% w/w) | 62 |
Syrup USP | 56 |
Glycerol (glycerin) | 46 |
Methanol | 33 |
Propylene glycol | 32.1 |
Ethanol | 25 |
n-Propyl alcohol | 22 |
Acetone | 21 |
Polyethylene glycol 400 | 12.4 |
Chloroform | 5 |
Castor oil | 4.6 |
Ethyl ether | 4.3 |
Sucrose | 3.3 |
Olive oil | 3.1 |
Sesame oil | 3.1 |
Benzene | 2.2 |
Carbon tetrachloride | 2.2 |
Octane | 1.9 |
Solvents may be classified according to their dielectric constants as polar (δ > 50), semi-polar (δ = 20 – 50), or non-polar (δ = 1 – 20).
The value of the dielectric constant for a mixture is obtained by multiplying the volume fraction of each solvent times its dielectric constant and summing.

There are many pharmaceutical substances which are non-polar or which are weak acids and bases whose ionized salt forms are unstable in solution. In order to dispense solutions of these substances, we must derive a solvent of appropriate polarity (or non-polarity).
Practically speaking, this is a fairly simple problem to solve. Solutions are prepared containing varying concentrations of ethanol or acetone in water, ranging from 0 to 100%. The required concentration of drug is added to each solution and the solutions are refrigerated overnight, then viewed for precipitation.
% v/v ETOH | 0 | 10 | 20 | 30 | 40 | 50 | 60 | 70 | 80 | 90 | 100 |
---|---|---|---|---|---|---|---|---|---|---|---|
Precipitation | + | + | + | + | + | + | – | – | – | – | – |
In our example, we see that at least 60% v/v ethanol is required to solubilize our drug. This leaves us with a problem since, from a flavoring standpoint, 60% ethanol is not considered pharmaceutically elegant. (Why?) We can now take this information and determine the dielectric constant of the solvent system which provides drug solubility.
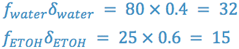
From this information it is possible to formulate a vehicle, substituting other solvents, which is of the necessary polarity and is pharmaceutically elegant.
These calculated values of δ are only approximate. Interactions between multiple solutes and solvents may increase or decrease solubility. Nonetheless, the use of the dielectric constant in formulating solvent systems gives us a simple and scientific approach to estimating our needs. It is, therefore, a useful tool.
e.g., Formulate a vehicle containing water, ethanol, and glycerin with a δ = 47. Limit ethanol to 20% by volume.

Thus, the vehicle is 20% v/v ethanol, 15% v/v water, and 65% v/v glycerin.